this operating point makes this
distortion substantially lower than is normally found with a conventional
average-area reader. Therefore a reference track of known dimension (the
control track) is recorded together with the audio information. The circuit
logic compares this reference pulse width to an internal record image scale
factor. Small errors generate a differential correction voltage, offsetting the
slicing point, for the lowest distortion. By this technique, high-quality
reproduction is ensured for transmittance variations resulting from normal
laboratory processing tolerances. Direct-current offset, or envelope
rectification voltages, frequently generated by speech sibilance, are reduced
together with their expansion during playback.
Normally, the
slicing level is close to 50% of the minimum/maximum transmittance when the
edge image projected on the CCD has an exponential or Gaussian
distribution, starting from the minimum transmittance region. Under these conditions,
the scaled CCD-derived pulse width will be proportional to the object image
size, with first-order independence from minor focus errors. At this level,
dirt having a lower transmittance and emulsion scratches having a greater
transmittance, if falling reasonably outside of the 50% transition zone, will
not reproduce as pulses and therefore as neither signal nor noise. Two sources
of noise remain: (1) very large particles of high optical density, or severe
emulsion scratches, will reproduce as noise, together with (2) anomalies
occurring within the transition zone. There is a low probability for the
occurrence of transition anomalies, where the noise duration is equal to the
ratio of the particle size in the transition zone to the full-channel track
width. Noises from these two sources reproduce with about the same intensity
as on conventional uncompanded Academy tracks, played on a normal reader. However,
it will be appreciated that the
statistical probability for the occurrence of both types of noise occurring is
very low.
The center slice
point is set as a ratio of illumination intensities, rather than an absolute
illuminance. Thus reasonable changes of the CCD illumination will alter the
slicing transmittance from which the pulse train is derived. Normal lamp aging
and the buildup of optical dirt will cause a slight noise increment, due to
less than optimum operation of the CCD. However, the more important signal
levels and crossmodulation settings will not drift.
Operation of the Scanner.
The Colortek system relies upon the usage of a commercial CCD
operating near its maximum useful clock frequency. The availability of this
device, at the present stale of the art makes the system practical. The 256-cell
buried-channel CCD has a practical maximum clocking and cell-to-cell shift rate
of about 10 MHz. This limit, which is imposed by using an optimum combination of
drive currents, illumination intensity requirements and operating dynamic range,
gives a 20-µs scan window. After adding the reset and scan start conditioning
signals, the overall cycle time is 25-µs yielding a 40-kHz scan rate. The complete
resulting waveform from the total scan of a dual bilateral track is shown in
Fig. 14 The upper information frequency limit which is restricted by filtering
and sampling methods, is normally at best 2.7 time less than the sample rate.
This gives maximum useful frequency response of 1 kHz. The scanned CCD slit is
17 µm wide and 3.3 mm long, which also makes the scaled useful
short-wavelength aperture limit correspond to 15 kHz, The cells are 13 µm
wide, allowing a 75% modulate quarter track signal with the necessary guard band scaling to sweep 30 of them. An expected peak
dynamic range of 46 dB gives an equivalent noise modulation of less than 1/3
cell, or 4 µm peak to peak.
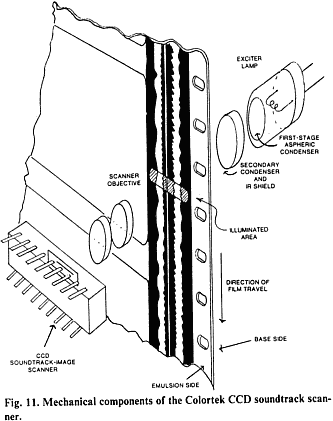 | 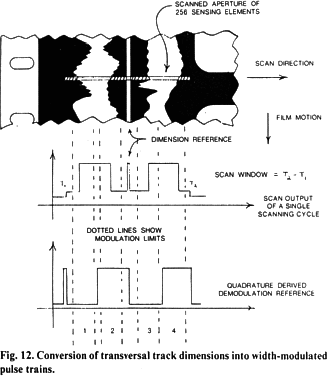 |